Team:Goettingen/Notebook/Results
From 2012.igem.org
![]() | |
Deutsch ![]() ![]() |
![]() |
Contents |
Homing coli: Engineering E. coli to become tracking dogs
The model organism Escherichia coli is naturally capable of sensing substances in its environment and consequently moves directionally towards these, a phenomenon known as chemotaxis. Here, we apply directed evolution to chemoreceptors by targeting five amino acid residues in the ligand binding site to enable E. coli to perceive novel substances. In order to investigate mobility and directed movement towards a substance, an effective mobility selection method using special "swimming plates" is designed. Additionally, we attempt to improve E. coli's swimming velocity by creating new parts derived from its own motility apparatus. Based on our selection system, we identify variants of chemoreceptors with new binding specificities in the mutant library. By these means, we aim to train the bacterium to detect new molecules such as tumor cell markers. Once having established E. coli as our "tracking dog", the possible applications in medicine but also to environmental issues are virtually countless.
#1 - Selection / Swimming
Fine tuning of the experimental setup
During the course of the experimental work it has been the task of group one to design and test the parameters for the optimal swimming and chemotaxis assays. Eventually, it was the goal to be able to select for fast E.coli cells that show a chemotactic behavior. Firstly, swimming is only possible in media with a low concentration of agar. After testing agar concentrations in a range between 0.2% - 1%, it turned out that 0.3% is the best concentration for fast E. coli cells. Lower concentrations were not appropriate due to a lack of solidity of the swimming-agar. Secondly, different nutrient compositions were tested. On the one hand, it was necessary to identify a convenient nutrient composition to trigger bacterial swimming. On the other hand, the nutrients in the media should enable E. coli cells to follow a chemoattractant.
Here, we focused on two different media: Tryptone-swimming agar for the induction of E. coli swimming after one day of incubation and M9-minimal agar for the observation of chemotaxis (Burkart et al. 1998; Farrel et al. 2005). M9-minimal agar did not support intense swimming. Instead, swimming was initially observed after 2 days. In order to allow chemotaxis on M9-minimal agar, the addition of L-methionine was required, as described by Adler, 1972. In comparison to the tryptone-swimming agar, chemotaxis experiments were much more reasonable with the defined M9-minimal agar leading to a high degree of reproduction. Thirdly, different stocksolutions of attractants in the Whatman paper were tested, including L-aspartate (10 mM), a mix of amino acids (0,2% w/v) and tryptone (0,5% w/v). These components were used to trigger chemotaxis of the bacteria. Here, we used L-aspartate in most experiments when we worked with the aspartate receptor Tar of E. coli. We adjusted the volume of all attractants to 100 µl, which was the maximal amount that could be soaked into the Whatman paper (2 cm X 2 cm in size). Furthermore, it proved beneficial to pipette the 100 µl attractant solution on the Whatman paper before the paper was placed onto the center of the semisolid agar. Moreover, the incubation temperature turned out to be a crucial parameter for swimming assays. It is known that long-term bacterial growth at 37°C leads to inhibition of flagella growth. Therefore, incubation of swimming assay Petri dishes was conducted at 33°C.
The fine-tuning of the experimental setup was closely related to the choice of our E. coli strains. Since we worked a lot with the tar-knock-out mutant (Baba et al. 2006) that was created in the background of the laboratory strain DH10B, we tested all our media and parameters with DH10B in the first place. But, soon we realized that laboratory strains were not developed to be the best swimmers and we figured that other strains might have better swimming characteristics. Therefore, the chemotaxis strains MG1655 and RP437 joined our collection. In addition, the E. coli laboratory strain BL21 showed better swimming properties than the laboratory strains DH10B or XL1 blue (Fig. 1).
Library selection assay
The main aim of our project was to “engineer E. coli to act as tracking dogs”. Seven substances were chosen as new attractants for our “tracking E. colis”: some were taken because of structural similarity to L-aspartate, the target of the tar receptor, others because of medical relevance and a third group was made up by just subjectively interesting chemicals. The first substance was D-aspartate, which is the enantiomer of L-aspartate. L-aspartate-4-benzyl-ester was chosen because of its structural similarity to L-aspartate. Another substance was 2-Ethyl-1-hexanol, which can be detected in the breath of lung cancer patients (Filipiak et al. 2009). The well known stimulant caffeine, the flavoring substance vanillin and geraniol, the smell of roses, were tested additionally. Finally, sodium cyclamate was chosen, an artificially produced sweetener.
The mutational library of the tar receptor, our receptor of choice, was produced by focus group #3 . Here, focus group 1 designed a selection system for the “tracking E. colis” (Fig. 2A).
In our system, the BL21 cells containing the mutagenesis library of the Tar receptor were dropped on 0.3 % tryptone agar plates with the attractant soaked into Whatman paper placed in the middle. The clones that moved the fastest towards the attractant and the reference strain were selected in three successive rounds and the plasmid DNA of single clones was sequenced (see results of group 3).
In figure 2B, exemplary pictures of a round of selection are shown. Swimming on tryptone agar could be observed every time after one overnight of incubation at 33 °C, but the observed halos at the different attractants varied in size. The halos had mainly a roundish shape and no direct chemotaxis effect towards the attractants could be identified. The reference strain, containing the parent plasmid of the mutagenesis library, also swam but never as fast as the cells containing the library. The observed halos of the BL21 strain with the library and the reference strain had the same size in the negative control where H2Odd was used instead of an attractant. Therefore, every attractant triggered a chemotactic effect in BL21 cells holding the mutagenesis library. After the third round of selection, the fastest clones were identified and their plasmid DNA was isolated and sequenced.
In order to investigate, whether the observed swimming behavior was related to the vector containing the mutagenized tar or the cells themselves, the isolated and sequenced plasmids were transformed into fresh BL21 cells and applied to a new swimming/chemotaxis assay. Additionally to the respective attractant, the effect of L-aspartate and H2Odd was evaluated. In general, the observed halos of the fresh transformed cells were smaller than the ones of the selected clones every time (Figure 2C). Thus, it can be concluded that the cells themselves were also selected for faster swimming abilities.
L-aspartate also was an attractant for nearly every clone and the halos were never reduced significantly in size when H2Odd was applied to the Whatman paper.
Separation Assay and RFP-interference
One of the aims of group one was to invent an assay that enables us to separate two strains from each other that have grown and swam together in a mixed culture. This would be especially beneficial for the determination of the effect of the promoter strength for a tar insert in a vector or to determine whether an attractant affects two different strains in a different way.
In the beginning, it was planned to conduct the separation of the strains via the strong overexpression of RFP of one strain whereas the other strain would not express RFP. The ratio between the red and the white colonies would give us information about the distribution of the different strains in one swimming halo. After the first round of cloning the RFP gene and the tar inserts were on different vectors containing different antibiotic resistances. Therefore, a separation via the resistance marker seemed to be more plausible.
The separation assay described in the methods section was conducted multiple times and in many different variations, but the achieved outcomes were contradictory (no data shown).
In some cases when the agar from the middle of the drop was cut out a much higher number of colonies of the non RFP expressing strain was counted, even though the strains were mixed in the same ratio and thus should be present in the same amount. It was suspected that the expression of RFP contributes to the optical density value measured at a wavelength of 600 nm. Consequently a much higher OD600 value is measured than is actually proportional to the number of cells in the culture. Some experiments were conducted to determine the influence of the RFP expression on the OD600 value. Because of the time limit no statistically significant values could be gained and the achieved data is not shown. Further experiments have to be conducted to determine the RFP interference (e.g. using a counting chamber) and in case of the separation assay one should probably refrain from utilizing RFP expressing strains.
References
- [1] [http://mic.sgmjournals.org/content/74/1/77.full.pdf Adler J. 1972. A Method for Measuring Chemotaxis and Use of the Method to Determine Optimum Conditions for Chemotaxis by Escherichia coli. J Gen Microbiol. 74(1):77-91 ]
- [2] [http://www.pnas.org/content/95/5/2568.short+html Burkart M., Toguchi A., Harshey R.M. 1998. The chemotaxis system, but not chemotaxis, is essential for swarming motility in Escherichia coli 3;95(5):2568-73 ]
- [3] [http://www.nature.com/nmeth/journal/v2/n5/full/nmeth0505-377.html Farrell I.S., Toroney R., Hazen J.L., Mehl R.A., Chin J.W. 2005. Photo-cross-linking interacting proteins with a genetically encoded benzophenone 2(5):377-84]
- [4] [http://ar.iiarjournals.org/content/29/1/419.full.pdf+html Filipiak W., Sporing A., Filipiak W., Mikoviny T., Ager C., Schubert J., Miekisch W., Amann A., and Troppmair J.. 2009. Release of Volatile Organic Compounds from the Lung Cancer Cell Line NCI-H2087 In Vitro. Anticancer Research 29: 419-426.]
#2 - Speed Improvement
The goal of our group was to enhance the motility of E. coli in order to allow them to reach their targets more efficiently in later applications. This also results in a a faster selection of our Homing Coli and quick generation of results. The BioBricks we designed are therefore primarily directed at flagellum efficiency or number. We selected five different genes that were tested along our project in different strains. E. coli strain BL21 was used as our main object of research because this is a typical lab-strain and therefore usually not very motile. Our goal was to find a way to accelerate BL21 E. coli by transforming them with our constructs. Here is an overview of the genes we tested and the corresponding results.
motA and motB
These genes code for proteins that build the stator part of the bacterial flagellum which generates torque by using a proton gradient that exists across the membrane (Fig. 3). Flagellum function depends on copy number of motA and motB (Van Way et al. 2000). Therefore we chose to test these genes, hoping that the number of stator protein copies might have a positive effect on motility (Reid et al. 2006). We first tested our strains with motA and motB constructs containing the natural 3’ and 5’ regions in puc18 because a strong overexpression might have counterproductive effects. High expressed motA might thereby lead to proton leakage and disturb the metabolism of E. coli.
- Results
- For these two stator-genes it was very hard to get reproducible data. However, after several rounds of testing on different swimming agars and conditions we were able to conclude that overexpression of motB often had a mild positive effect on motility of BL21 E. coli (Fig. 4). However, increased expression on motA seemed to have no or even a slightly negative effect.
- We did not see very strong effects after testing these two candidates. A possible explanation could be that the number of functional stator elements at one flagellum could not be increased anymore or might just not have a significant impact. Furthermore the expression of motA and motB is usually regulated in concert and if out of balance, their expression might not cause any positive effect for motility. For future projects it might be interesting to test chimeric stator proteins that could harbor higher efficiencies or use other ions for torque generation.
yhjH
This gene codes for a phosphodiesterase which reduces the levels of c-di-GMP that are involved in cell motility (Ko and Park, 2000). The protein YcgR is able to bind c-di-GMP and act as a flagellar brake which consequently reduces motility (Paul et al. 2010). The mechanism that we would like to exploit is illustrated in Figure 5. Overexpression of yhjH should therefore result in lower levels of c-di-GMP and increase E. coli's speed by diminishing the ability of YcgR to act as a brake on the flagellum. The second messenger c-di-GMP furthermore plays a role in the regulation of biofilm development. Expression of yhjH on the other hand represses biofilm formation (Suzuki et al. 2006) which could also serve our purposes and induce swimming motility.
![]() Fig. 5: The yhjH gene encodes for a c-di-GMP phosphodiesterase. The substrate, c-di-GMP, is a second messenger that binds to YcgR, a protein that functions as a flagellar brake and thus down-regulates the motor (A). We suggest that the overexpression of yhjH results in increased c-di-GMP degradation and hence in narrowed braking force. Due to a stronger rotation of the flagellum higher motility could be achieved (B). References: http://www.uniprot.org/uniprot/P37646; http://www.uniprot.org/uniprot/P76010 | ![]() Fig. 6: BL21 E. coli carrying different constructs on Tryptone swimming agar after 12h incubation at 33°C. Cells expressing yhjH in puc18 under the natural promoter travelled approximately 0.5cm (radius) whereas no swimming could be detected for the control plasmid carrying [http://partsregistry.org/Part:BBa_K777125 K777125]. |
- Results:
- Expression of yhjH under its natural promoter in puc18 increased motility in almost all of our assays on LB or Tryptone swimming agar (Fig. 4 & Fig. 6). Not only were the yhjH expressing strains among the fastest on these swimming agar plates but also usually the first colonies to start swimming. This might be an indicator that indeed biofilm formation was inhibited and the E. coli were able to spread faster. Compared to our other tested constructs we got the most reliable results for yhjH. However the positive effect on motility was not present when we used M9 minimal medium swimming agar. Here, yhjH-transformed cells usually did not show a strong tendency for swimming. This result suggests that the motility-effects of YhjH are somehow nutrient-dependent and therefore depend on the used media.
fliC
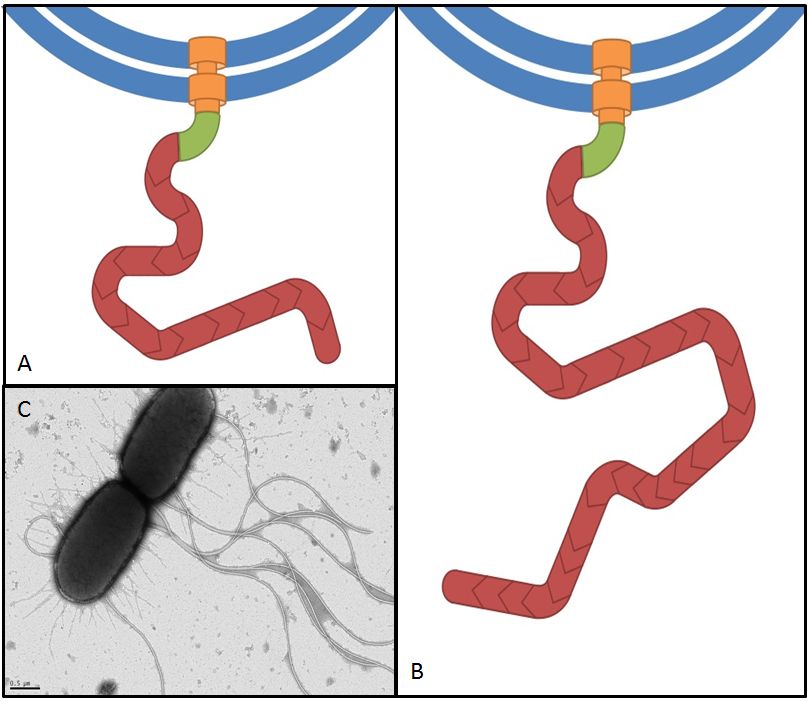
References:
http://ecocyc.org/ECOLI/NEW-IMAGE?type=POLYPEPTIDE&object=EG10321-MONOMER
Flagellin, the product of the fliC gene is the structural protein that builds up the filament of the bacterial flagellum (Fig. 7). By overexpressing fliC we hope to construct longer flagella which might have a positive impact on swimming speed (Furuo et al. 1997). At the same time we were aware that overlong flagella might cause adverse effects due to an obstructive architecture.
- The fliC gene was amplified from DH10B E. coli genomic DNA and contained four forbidden restriction sites. We had to mutate all these sites via overlap PCR. A description for this method can be found here.
- Results
- Our overlap PCR for the mutation of three PstI and one SpeI sites worked efficiently and fast. The resulting PCR product was not cut by any BioBrick standard 10 enzymes.
- In order to avoid too strong levels of expression we included 1kb of the upstream region for most of the assays to allow a certain level of regulation in the cells. Like yhjH-transformants these strains showed increased motility in almost all of our assays (Fig. 6). On the minimal medium M9 agar plates they were usually significantly faster and showed swimming motility earlier than any other cells. On Tryptone swimming agar they were in general the runner up behind yhjH-transformed E. coli. Unfortunately, we had trouble cloning our fliC constructs into pSB1C3 because of a problem in our reverse primer suffix. When we had located the source of the problem it was too late to send this part to the Registry in time.
flhDC
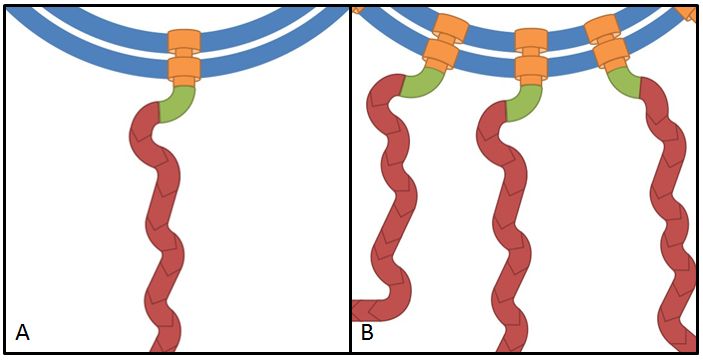
References:
[1] Wei et al. (2001) Positive regulation of motility and flhDC expression by the RNA-binding protein CsrA of Escherichia coli. Molecular Microbiology, 40(1):245-256
[2] Gauger et al. (2007) Role of motility and the flhDC operon in Escherichia coli MG1655 colonization of the mouse intestine. Infect immune 75(7):3315-3324
The flhDC operon is the master regulator of motility and chemotaxis in E. coli. This means that it is the main control instance for flagellar synthesis and starts the complex process of flagellar gene synthesis and flagellum assembly (Chevance and Hughes 2008). The flhDC operon codes for the transcriptional regulator FlhD4C2 which forms heterotetramers and activates class II operons in concert with sigma factor 70. Among the gene products of class II operons are several components of the flagellum and the alternative sigma factor FliA which is essential for the transcription of class III genes. It has been shown that increased expression of flhDC also enhances motility in E. coli (Ling et al. 2010). We wanted to see how different levels of flhDC expression affect the speed of our E. coli lab-strains. In theory, the number of assembled flagellums should increase (Fig. 8) and thereby have a positive impact on motility.
- Results
- Our plan was to express flhDC constitutively with a selection of 8 different Anderson-promoters to achieve different levels of transcription. And indeed BL21 E. coli transformed with these constructs showed a higher motility than empty vector controls or WT cells. However, our results were very inconsistent, especially concerning the different promoter strengths when compared to each other. The troubleshooting revealed that we were missing a RBS between the promoters and the ATG within all our constructs. This probably explains the inconsistent results that did not allow us to quantify the effect of our constructs. We hope to finish these experiments with correct constructs soon because we are eager for presentable results!
Electron microscopy
Overexpression of flagellar genes like fliC or the flhDC operon should result in alterations of the flagellum structure or number, which can be visualized. In the case of fliC, which encodes flagellin, the building block of the flagellum, we expect the emergence of longer filaments. In contrast to this, flhDC as the master regulator is thought to increase the number of flagella when overexpressed, since all genes involved in flagellum assembly should be up-regulated.
In order to visualize these alterations we investigated the E. coli strain BL21, transformed with fliC or flhDC constructs, by using transmission electron microscopy. Both genes were under control of a strong constitutive promoter promoter (J23100). As reference strains we used BL21 cells carrying the same vector with an RFP insert. Furthermore the MG1655 strain, as a more motile strain with non-degenerated flagella, was used.
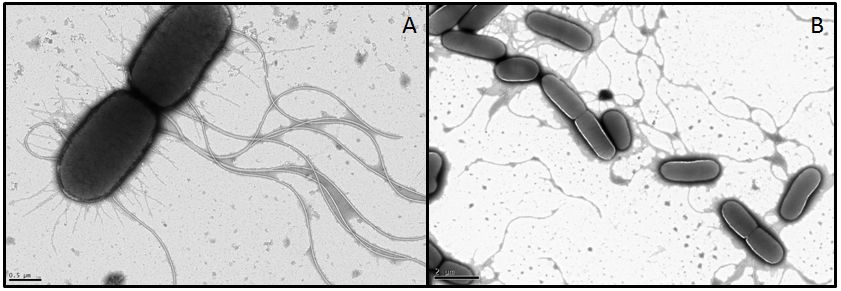
- Results
- For MG1655 nice pictures were obtained since the strain grew very fast and seems to be quite robust (Fig. 9). However, we were not able to obtain meaningful pictures showing flagella of the BL21 strain. Under stress conditions bacteria tend to lose their flagella very easily (Blum, 1971). Apparently the transport form our Lab to the EM-facility was enough to stress the BL21 E. coli. It appears that this demands quite something for MG1655 however, or the pictures we got from this strain were already in a stressed state and the flagella are usually even longer or more in number. We had a look at the different strains with a light microscope in our lab before the EM sessions and they were all motile. This consideration, that the cells were stressed during the transport was supported by their subsequent investigation under a light microscope. The cells were not motile anymore and featured very heterogeneous shapes whereby some formed very long rods. All these appearances are indications for stressed cells. Another problem with BL21 was the very low growth rate, since a high optical density is required in order to obtain an adequate number of cells during preparation. Therefore we had to centrifuge the cultures in some cases which ended up in interwoven filaments (Fig. 9).
- In conclusion, we were not able to get informative data about the flagellar structures and morphologies, but we found out, that BL21 is very sensitive and drops its flagella in no time.
- For MG1655 nice pictures were obtained since the strain grew very fast and seems to be quite robust (Fig. 9). However, we were not able to obtain meaningful pictures showing flagella of the BL21 strain. Under stress conditions bacteria tend to lose their flagella very easily (Blum, 1971). Apparently the transport form our Lab to the EM-facility was enough to stress the BL21 E. coli. It appears that this demands quite something for MG1655 however, or the pictures we got from this strain were already in a stressed state and the flagella are usually even longer or more in number. We had a look at the different strains with a light microscope in our lab before the EM sessions and they were all motile. This consideration, that the cells were stressed during the transport was supported by their subsequent investigation under a light microscope. The cells were not motile anymore and featured very heterogeneous shapes whereby some formed very long rods. All these appearances are indications for stressed cells. Another problem with BL21 was the very low growth rate, since a high optical density is required in order to obtain an adequate number of cells during preparation. Therefore we had to centrifuge the cultures in some cases which ended up in interwoven filaments (Fig. 9).
References
- Blum J.J.(1971) Existence of a breaking point in cilia and flagella. J. Theor. Biol. 33:257–263.
- Chevance F. F., Hughes K. T. 2008. Coordinating assembly of a bacterial macromolecular machine. Nat Rev Microbiol. 6: 455–465.
- Furuno, M., T. Atsumi, T. Yamada, S. Kojima, N. Nishioka, I. Kawagishi, and M. Homma. 1997. Characterization of polar-flagellar-length mutants in Vibrio alginolyticus. Microbiology. 66: 3632–3636.
- Ko M., Park C. 2000. Two novel flagellar components and H-NS are involved in the motor function of Escherichia coli. J. Mol. Biol. 303: 371-382.
- Ling H., Kang A., Tan M.H., Qi X., Chang M.W. 2010. The absence of the luxS gene Increases swimming motility and flagella synthesis in Escherichia coli K12. Biochem Biophys Res Commun. 401: 521-526.
- Paul, K., Nieto, V., Carlquist, W.C., Blair, D.F., Harshey, R.M. The c-di-GMP Binding Protein YcgR Controls Flagellar Motor Direction and Speed to Affect Chemotaxis by a “Backstop Brake” Mechanism. 2012. Mol Cell. 38: 128–139.
- Reid, S. W., M. C. Leake, J. H. Chandler, C. J. Lo, J. P. Armitage, and R. M. Berry. 2006. The maximum number of torque-generating units in the flagellar motor of Escherichia coli is at least 11. Proc. Natl. Acad. Sci. USA 103: 8066-8071.
- Suzuki, K., Babitzke, P., Kushner, S. R., Romeo, T. 2006. Identification of a novel regulatory protein (CsrD) that targets the global regulatory RNAs CsrB and CsrC for degradation by RNase E. Genes Dev. 20: 2605–2617.
- Van Way, S. M., E. R. Hosking, T. F. Braun, and M. D. Manson. 2000. Mot protein assembly into the bacterial flagellum: a model based on mutational analysis of the motB gene. J. Mol. Biol. 297: 7-24.
#3 - Chemoreceptor Library
Prologue
The objective of the “Legendary TAR librarians” was the generation of a mutant library of the chemoreceptor Tar (Taxis to Aspartate and Repellants). Tar is one of five membrane-bound chemoreceptors E. coli uses for chemotaxis [1]. The receptor is well characterized and an X-ray structure is available (see [http://www.rcsb.org/pdb/explore/explore.do?structureId=1wat1 PDB file]). Residues of the Tar ligand-binding domain that interact with L-aspartate were identified from the pdb-file. Here, we aimed to identify novel receptors by applying directed evolution to Tar chemoreceptors. To enable E. coli to perceive novel substances five amino acid residues in the ligand binding site were the target. Our method of choice is targeted saturation mutagenesis. In comparison to random mutagenesis, this has the advantage to specifically mutate the selected amino acid residues.
Chapter I: Preparing Tar for the Library
The amplification of Tar was carried out directly from the E. coli K12 genome. We introduced the BioBrick standard restriction sites by primer design (see sequencing primers; Tar for + prefix & Tar rev + suffix). The amplified BioBrick part was then inserted into the vector pUC18. To get rid of BioBrick standard restriction sites in tar, a QuikChange reaction was applied. The XbaI site (nt 420-425) was exchanged from TCTAGt to CCTAGt while keeping the codon for the same amino acid. Our next step was to express the quikchanged tar (TAR_QC) under the constitutive [http://partsregistry.org/wiki/index.php?title=Part:BBa_K777001 Anderson promoters].

With these various promoter constructs in hand, a swimming assay was performed. This experiment revealed promoter J23100_TAR_QC in pUC18 background giving the best swimming abilities (data not shown). To generate a chemoreceptor library our method of choice requires the absence of the BsaI restriction site in both, the insert and the vector backbone, of the cloned plasmid. Since pUC18 contains a BsaI restriction site in the plasmid backbone within the selection marker bla gene, this vector is not appropriate for targeted saturation mutagenesis. Thus, the construct was cloned in the BioBrick vector pSB1C3.
The promoter constructs in the new vector backbone were tested in chemotaxis assays for swimming towards L-aspartate (Fig. 10). Thereby, all eight tested promoter constructs were dropped around in the same distance to an octahedron Whatman paper soaked with L-aspartate. As a control serves the strain BL21 transformed with Tar_QC under the control of the eight promoters. ∆Tar was complemented with Tar_QC. As expected, the strongest promoters (18C = K777001, 18K = K777002) show the strongest movement. It was not possible to evaluate 18O (K777004). All in all, the complementation worked, but not to the expected extend.
Further proof came from a SDS-PAGE using where we observed an additional protein band in lysates from cells producing tar from one of three strongest Anderson promoters. The results were consistent with the previous swimming study (data not shown). The construct J23100_TAR_QC gave the best results in these experiments and was therefore used in all further experiments.
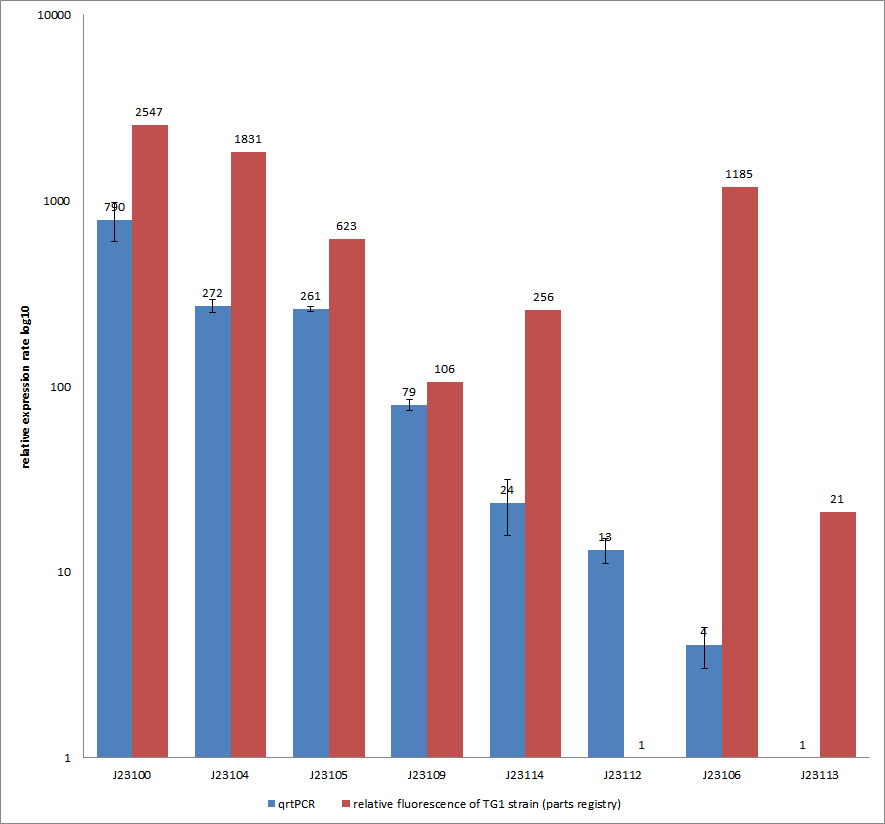
Additionally, our goal was to see a correlation between the mRNA expression levels and the motility of our engineered E. coli on swimming plates. Therefore, we used quantitative real-time PCR (qrtPCR) as a powerful tool for quantification of gene expression. We used this method to examine the expression rate of the eight different constitutive promoter constructs from the parts registry that were further confirmed by swimming assays. The reported activities of these promoters are given as the relative fluorescence of these plasmids in strain TG1 (parts registry: [http://partsregistry.org/Part:BBa_J23100 Part:BBa_J23100]). The results are depicted in Figure 11. Here, promoter constructs were cloned into the vector pSB1C3 and expressed in E.coli BL21DE3 grown in LB-media (lysogeny broth). The measurements were performed as a triplet for each construct and a house-keeping gene coding for a 16SrRNA (rrsD) as reference. Additionally, we included H2O as negative no template control to predict possible contaminations. For the evaluation of our results, the 2–ΔΔCT (Livak) method was applied. We used the weakest promoter with the lowest expression rate as calibration for the calculations and as reference the housekeeping gene. The data was then compared to the predetermined expression rates from iGEM2006_Berkeley group.
Overall, as shown in Figure 11, each tested promoter construct indicated differences in expression rates in comparison to values from the parts registry. In fact, both data-sets were collected by methods which produce data at different points after gene expression. A common trend was detected for the strongest promoters J23100, J23104, J23105 and J23109 together with the weakest promoters J23112 and J23113. Conspicuously, the promoter J23109 revealed for qrtPCRand for relative fluorescence measurements nearly the same expression rates. The expression rates of J23114 and J23106 indicated massive differences in their expression rates and no common trend with the expression values from the parts registry. We detected comparable positioning in the ranking of expression rates (see characterization of constitutive promoters by qrtPCR) for six out of our eight promoters. The promoters characterized as relatively strong were also, in our case, responsible for higher expression rates and the other way around. In the case of promoter J23114 and J23106, the data-sets exhibited a completely different characterization compared to those in the parts registry.
All in all, these results confirm the properties of J23100 as the best swimmer.
Chapter II: Designing the Library
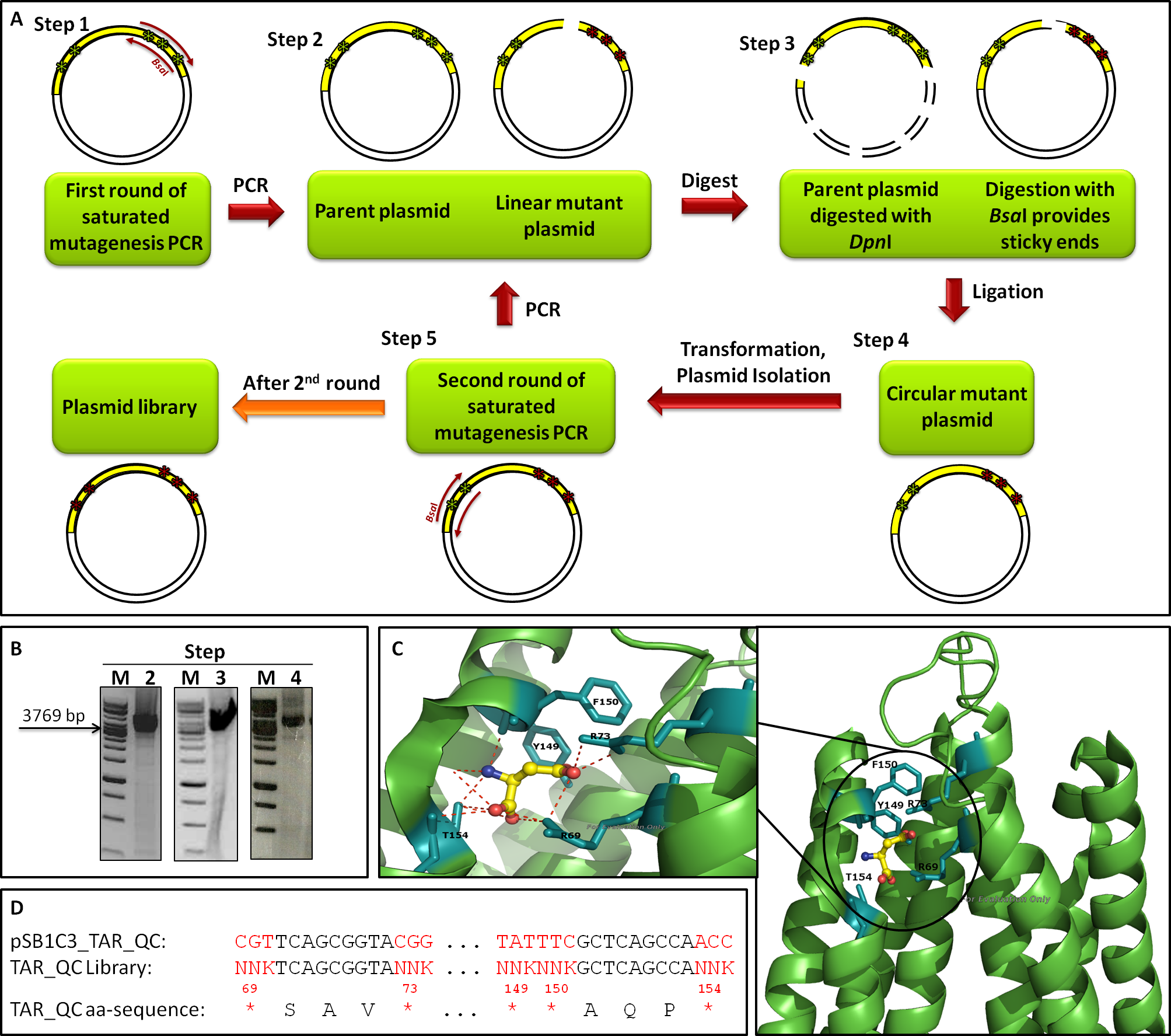
The introduction of targeted saturation mutations requires mutant primer design (primer mutagenesis). We divided our library generation into two rounds, each mutating different residues (Fig. 12A). Amino acids 149, 150 and 154 were mutated in the first (1st), amino acids 69 and 73 in the second round (2nd). Each library generation consists of the following steps: 1. primer design, 2. saturated mutagenesis PCR, 3. DpnI/BsaI restriction, 4. ligation, transformation and plasmid isolation, 5. PCR for 2nd round; the template for 2nd round being the isolated mutated plasmids of the 1st. After 2nd round, the plasmid library is completed. Figure 12B shows gels from the 2nd round of mutagenesis. The gels of step 2 (PCR), step 3 (digest) and step 4 (ligation) show a band of the expected size at 3769 bp, indicating that the intermediate steps were successful. The primers we used for the mutagenesis PCR randomly mutate five amino acids residues at positions 69, 73, 149, 150 and 154, which are known to bind to L-Aspartate (Fig. 12C). The outcome of the mutations is depicted in Figure 12D.
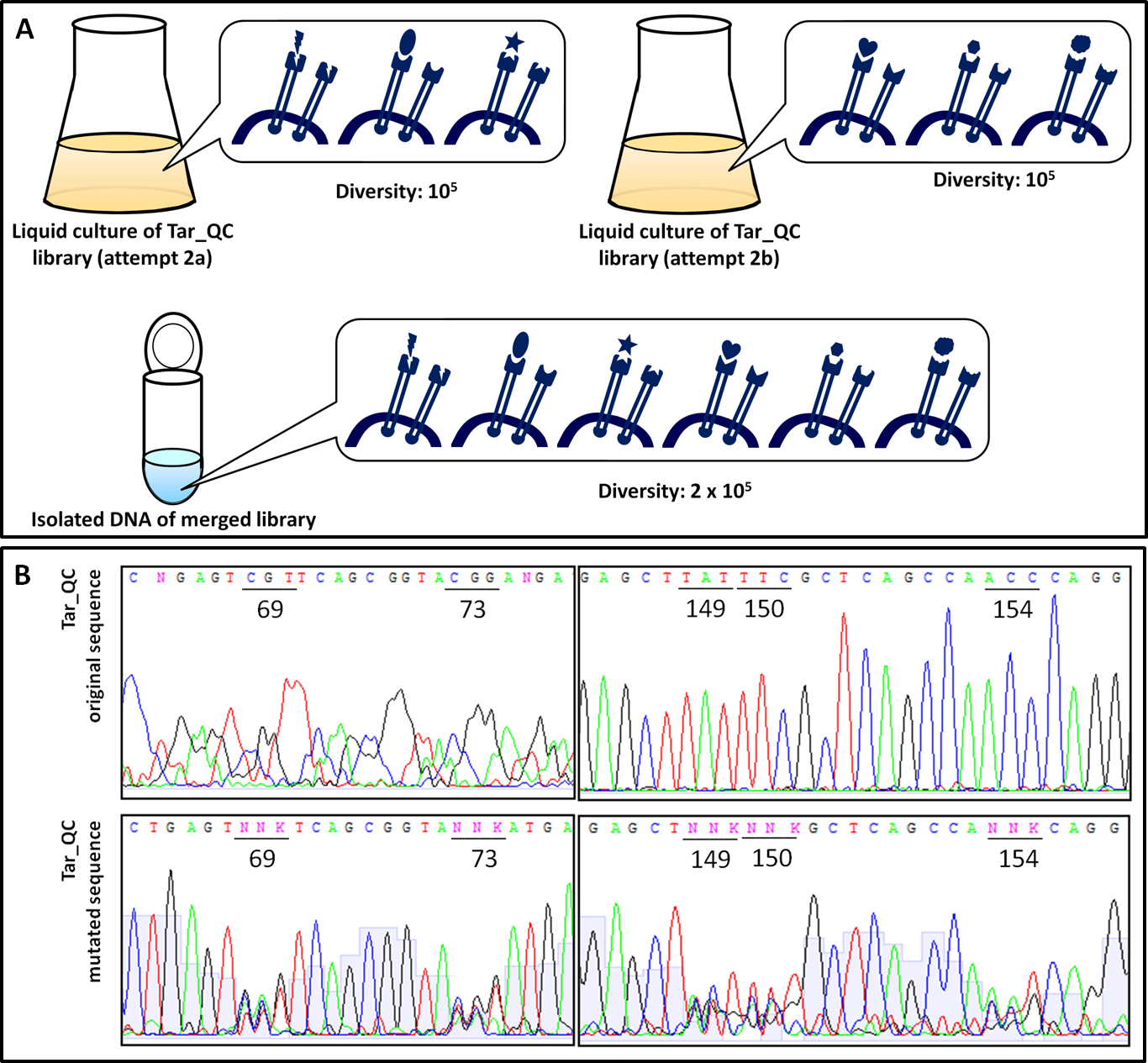
In order to examine the property of the generated chemoreceptor J23100_TAR_QClibrary the transformation efficiency was determined. Due to the fact that the first liquid culture of TAR_QC library only revealed 105 clones (attempt a), a repeat of the 2nd round of the library (attempt b) was carried out to increase diversity and resulted in the same diversity. Isolated DNA of attempts a and b were merged to gain a final diversity of 2x105, covering 6% of the theoretical diversity which would be 3.26 (Fig. 13A). The comparison of the sequencing chromatograms between the original and the mutated sequence excerpts indicated high quality of the TAR_QC library, because only the expected mutation sites had been changed. Every sequence of the isolated liquid culture DNA, which was not targeted, remained the same (Fig. 13B).
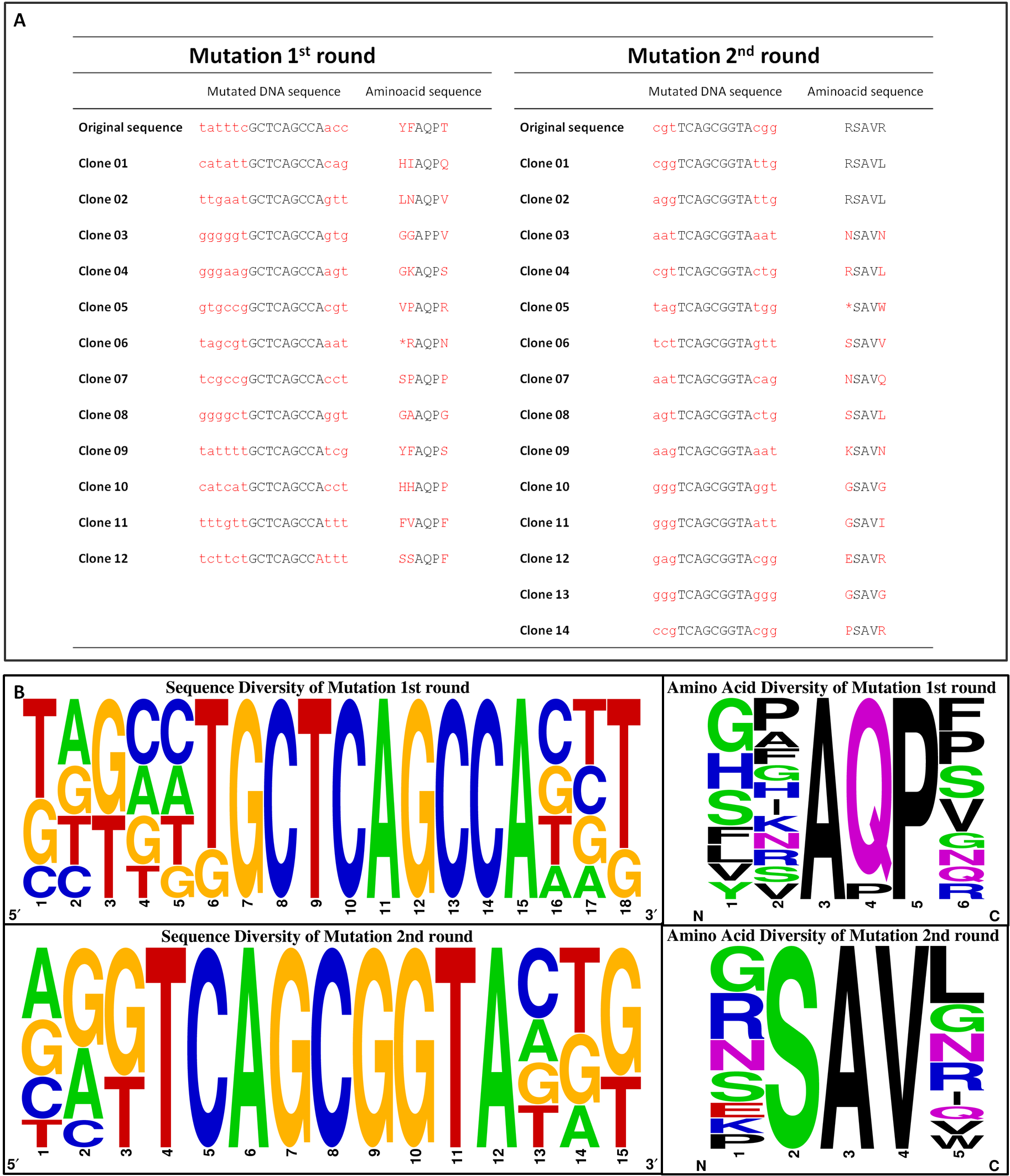
To exclude the possibility of a bias towards specific amino acids within the library, clones of the transformation efficiency plates were picked randomly for sequencing. The nucleotide and corresponding amino acid sequence are represented in Figure 14A. No clones were picked twice and only two deletions/frameshifts were identified. On the basis of these data the sequencing alignment was done. Figure 14B supports the good quality of the generated library showing the DNA sequence and amino acid diversity. The sequence alignment also reflects the well-targeted mutations as conserved nucleotides are resembled according to their letter size. As shown in Figure 12D, the used mutation primers incorporated NNK at desired positions, whereby N stands for any possible nucleotide and K for guanine or thymine. All introduced nucleotides ‘N’ show at least three diverse nucleotides, whereas all introduced nucleotides ‘K’ showed the expected pattern. The diversity of the nucleotides is reflected in the translated amino acid sequence.
To sum up, our J23100_TAR_QC library is unbiased, well targeted and of good quality.
Chapter III: Reading the Library
Based on the knowledge of the well characterized J23100_TAR_QC library, we assumed to have constructed novel chemoreceptors. Due to the modification of the binding site these should be capable of recognizing new chemical substances. Therefore, we performed library selection assays using seven different chemicals. We expected E. coli to perform chemotaxis towards these chemicals as illustrated in Figure 15.
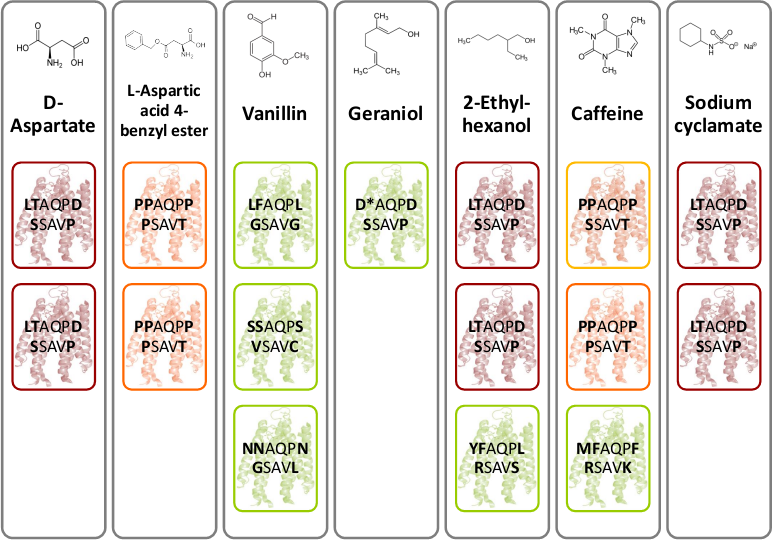
D-aspartate and L-aspartic acid-4-benzyl ester were chosen because of their structural similarity to D- aspartate. Moreover, the four flavors – vanillin, geraniol, caffeine and sodium cyclamate were interesting to test. The most interesting chemical is 2-ethyl-hexanol, a volatile organic compound related to lung cancer [2]. As mentioned above, the library selection assay was performed (Fig. 2A). After three rounds of selection of the fastest E. coli were sequenced. Figure 16 depicts novel identified chemoreceptors. A particular color code reflects the sequence identity and structure similarity. The novel receptor colored in red with amino acid sequence LTAQPD…SSAVP was identified two times for D-aspartate, 2-ethyl-hexanol and sodium cyclamate. Another novel receptor colored in orange with amino acid sequence PPAQPP…PSAVT was found two times for L-aspartic acid-4-benzyl ester and once for caffeine. However, for caffeine a structural related receptor mutant to the orange colored receptor was identified since the amino acids proline and serine are similar in structure.
In conclusion the selection method worked although the selectivity was low. Nevertheless, we hold two novel chemoreceptors in our hands. All these experiments provide evidence for the functionality of the BioBrick K777001.
References
- [1] [http://chemotaxis.biology.utah.edu/Parkinson_Lab/publications/PDFs/Hazelbauer%20et%20al.,%202008.pdf Hazelbauer G.L., Falke J. J., and Parkinson J. S. 2008. Bacterial chemoreceptors: high-performance signaling in networked arrays. Trends Bichem Sci. 33(1): 9–19.]
- [2] [http://ar.iiarjournals.org/content/29/1/419.full.pdf+html Filipiak W., Sporing A., Filipiak W., Mikoviny T., Ager C., Schubert J., Miekisch W., Amann A., and Troppmair J.. 2009. Release of Volatile Organic Compounds from the Lung Cancer Cell Line NCI-H2087 In Vitro. Anticancer Research 29: 419-426.]
↑ Back to top! |
![]() |